The automotive competition sector is a unique industry with very demanding and complex requirements. Vehicles often require bespoke parts designed for one-off prototypes or short production runs. These components must meet stringent strength, stiffness and weight standards and be delivered to very tight deadlines. In addition, these vehicles are often unique and require redesign each season.
The demanding characteristics of this sector might suggest that technologies such as LMD are out of the question.
However, traditional methods, such as machining or welding, are limited in their ability to efficiently produce complex geometries. This creates a perfect opportunity for innovative approaches such as hybrid manufacturing to shine, in this case using Meltio’s 3D printing technology solution, the Meltio M450.
In this blog, we will see how Hirudi, our sales partner, has chosen Meltio’s WLM-DED technology to demonstrate its potential, not only in this sector but also in other sectors where complex geometries are required.
1. Application Overview: Key Features and Description
The Meltio system was tested on a critical and very demanding component of the suspension system of a mountain climb racing car prototype that has been selected, known as a Stub axle.
This part plays a vital role in transferring forces between the wheel and the chassis. These forces are generated in the contact track between the wheel and the ground through the suspension arms, ensuring optimal handling and safety.
2. The Must-Have Requirements for the Part
Based on the geometric and operational conditions, a design volume is defined to ensure adequate anchorage and accessibility for tools.
The main objectives are strength, stiffness and lightness, balanced by iterations that validate the design. Hybrid manufacturing combines traditional techniques with technologies such as 3D printing, enabling complex geometries and material optimization.
This approach opens new doors to more efficient, sustainable and advanced components.
3. Essential Conditions for Efficient Part Manufacturing
The design of the stub axle for hybrid manufacturing represents a breakthrough in exploring new possibilities in automotive engineering. Initially, the design focused on boiler-making and machining processes, leveraging their advantages while minimizing limitations and costs.
The development began with a framework of geometric and operational conditions set by other vehicle systems, along with mechanical strength requirements and objectives for stiffness and lightness. These geometric and operational conditions define the design’s volume, establish anchorage points for other components, and ensure accessibility for tools and part installation.
Meeting mechanical requirements involves an iterative process to verify compliance with strength and stiffness criteria, ensuring the component meets all functional and structural demands.
4. Key Objectives
The objective of this project was to explore the possibilities of the concept of hybrid manufacturing (additive + subtractive) for parts with certain relevance to the industrial fabric.
Achieving a lightweight yet robust design: Exploit the potential of the Meltio system to solve geometric challenges and design a rigid and lightweight part.
Reducing waste and machining time: Reduce the cost of this element with respect to the SLM alternative by improving the metallurgical quality while maintaining a certain geometric complexity.
Minimizing constraints: Reduce delivery time and costs compared to other manufacturing processes.
5. Exploring the Phases: A Case Study Breakdown
The design process begins with an understanding of the conditioning factors imposed by the Meltio M450 system, which governs the additive manufacturing and subsequent machining processes. These conditioning factors can be categorized into 2 main types:
1. Work Volume Limitations:
The physical size of the workpiece is limited by the maximum work envelope of the system, which is 150 × 200 × 450 mm. This limitation directly affects the dimensions of the part that can be produced.
2. Design Guidelines for Optimal Printing:
The Meltio M450 system has a set of recommended values for various geometric features to ensure optimal print quality. These guidelines define best practices for design elements such as wall thickness, overhangs, and unsupported features that contribute to the stability and integrity of the printed part.
By thoroughly studying these guidelines, designers can ensure that the parts created align with the capabilities and limitations of the Meltio M450, leading to efficient and high-quality manufacturing outcomes.
TIP: Discover our 3D Printing guidelines by downloading the Meltio Design Guidelines
5.1. Part Analysis: From Conception to Creation
The Stub Axle was designed after analyzing the factors defining its functional and manufacturing requirements.
The process began by adapting the design to suit Meltio’s additive capabilities and subsequent machining. The Stub Axle’s geometry includes elements that efficiently absorb and transmit external loads (bearings, spherical plain bearings, bolted joints, etc.) and another series of geometric elements whose purpose is to transmit the load between the former.
Self-supporting geometries were prioritized to minimize the need for additional supports during printing, reducing material usage and manufacturing time. With the use of the Meltio system, the design maximizes mechanical efficiency by optimizing material placement specifically in high-stress regions.
This approach ensures mechanical efficiency, enhances durability, and reduces waste.
5.2. Optimizing Manufacturing: The Role of Iterative Refinement
The team refined the part’s design through multiple cycles of iteration, focusing on key factors like wall thickness, overhang angles, and material density to meet both performance and manufacturability goals. Supports were integrated into the CAD model to minimize machining effort and material use, making challenging areas self-supporting and eliminating the need for large external supports.
During manufacturing, process parameters were fine-tuned to optimize settings and reduce geometric limitations. The part was divided into fragments based on critical features affecting production.
The separation of the part into fragments was based on key geometric characteristics affecting manufacturing:
Size of isolated surface sections (islands): Parameters were fine-tuned to control energy and material flow on the surface, preventing overheating during printing.
Overhang angle: Adjustments ensured stability and precision in areas with substantial overhangs.
Path overlapping: Optimization of path overlaps ensured proper material consolidation for structural integrity.
Each fragment was carefully integrated, resulting in a smooth and robust final part.
5.3. Characterizing the Manufacturing Process
The results of the tests conducted yielded the following laminate configuration. Each section of the piece represents a distinct process where variables such as layer height, the number of perimeters, energy deposition, and contribution rate were adjusted.
The experiments pushed the limits of certain parameters, including cantilever angles, wall thicknesses, and the minimum manufacturable cross-section. This approach helped identify the constraints the design team had to address to ensure successful manufacturing.
Once these limits were identified, the necessary design adjustments were made. Full-scale manufacturing was then carried out, guided by the empirical findings from the tests.
Additionally, the hybrid approach enabled the fabrication of complex geometries that would have been difficult or impossible to achieve with traditional methods. This capability not only underscores the versatility of Meltio’s system but also demonstrates its scalability for a broad range of applications.
5.4. Simulation-Based Validation (FEA)
Finite Element Analysis (FEA) is an essential tool in designing high-speed racing components, allowing engineers to simulate real-world conditions and validate designs against lateral and longitudinal loads before manufacturing.
Each design iteration is tested using FEA to ensure mechanical integrity, even when adjustments focus on specific areas of the component. However, the entire part must be redesigned for a comprehensive analysis, ensuring all parts meet the required performance standards.
The material selected, ER70S6 mild steel, is chosen for its excellent weldability and ease of machining, making it suitable for high-precision, performance-driven applications. A mesh convergence study is conducted to determine the optimal element size that accurately captures the model’s complex geometry while ensuring efficient simulations.
Lateral Load Case and longitudinal braking loads
The primary load cases in the analysis include lateral and longitudinal braking loads.
The lateral load case simulates a 2G lateral acceleration, with safety factors applied to both horizontal and vertical loads to protect the suspension system while allowing suspension arms to yield under excessive load.
Based on a 2.5G braking acceleration, also incorporates vertical and horizontal forces, with safety factors applied accordingly.
(The analysis does not account for auxiliary components like bearings and joints, which contribute additional rigidity in the actual system. Overall, FEA ensures that components are designed to meet the extreme demands of high-speed racing while maintaining safety and performance.
6. Machining
The machining process was always present during the design development due to the constraints it imposes. It is only once the development is completed that the manufacturing plans are created to execute the machining. The parts to be machined were limited to the areas where the commercial components would be assembled, as well as the areas where supports were added during the design phase.
7. Impressive results
The results of the Meltio hybrid process speak volumes about its potential:
This advancement not only underscores the flexibility of Meltio’s system but also demonstrates its scalability for a wider range of applications.
8. Future possibilities for a greater impact
Titanium applications: Using titanium in the Meltio process could further reduce weight and machining costs, especially for high-performance applications.
Robotic integration: By using the Meltio Engine Robot, we would enhance geometric freedom, allowing for even greater material optimization and design flexibility. This integration could pave the way for autonomous, large-scale manufacturing tailored to automotive sector and other industries.
9. Conclusions
The aim of this project was to demonstrate how the Meltio system could meet the complex requirements of the part while reducing production costs and time. By combining the strengths of additive and subtractive techniques, it has delivered lightweight, high-performance parts faster and more cost-effectively.
We have streamlined production, tackled complex geometries, improved performance and took full advantage of the benefits of Meltio additive manufacturing.
The geometric limits of the 3-axis LMD system have been surpassed, allowing the technology to penetrate new markets. The Meltio three-axis system has been shown to be fully valid for the production of lightweight structural parts.
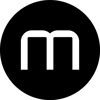
Written by Meltio
Meltio takes metal 3D printing to the next level by developing high-performance, affordable, and easy-to-use metal 3D printing solutions.